An Action Potential is Self Regenerating Because _____________
I’ ve spent countless hours studying the complexities of neuroscience, and one concept that never fails to intrigue me is the self-regenerating nature of an action potential. It’s a fundamental principle in neurobiology, driving everything from our most basic reflexes to our highest cognitive functions.
An action potential is self-regenerating due to its all-or-none response mechanism. In simpler terms, once this electrical impulse gets going, there’s no stopping it until it reaches its destination. The domino effect begins when a threshold level of depolarization occurs in a neuron. This causes voltage-gated sodium channels to open, resulting in an influx of positive ions into the cell and creating the initial spike that characterizes the action potential.
The beauty of this system lies within its refractory periods, which prevent backward propagation and ensure that each action potential remains a distinct event. This intricate dance between triggering stimulus and cellular response ensures reliable transmission of signals across vast neural networks – truly a marvel worth appreciating!
What is an Action Potential?
Diving straight into the heart of neuroscience, let’s unravel the mystery of what an action potential really is. In essence, it’s a change in electrical charge across a neuron’s membrane that sparks off a nerve impulse. This impulse travels down the fiber-like extensions called axons, delivering messages from one part of our body to another.
Imagine your body as a vast communication network. Your brain sends out orders and your muscles respond by moving, all thanks to these tiny electrical impulses known as action potentials. They’re like biological text messages zipping through your nerves at lightning speed.
To get technical for just a moment, action potentials occur when there’s a rapid switch in voltage across the cell membrane. At rest, neurons have what we call ‘resting potential’, with more negative ions inside than outside their cell membranes. But when triggered by stimuli (like touch or smell), ion channels on the neuron open up.
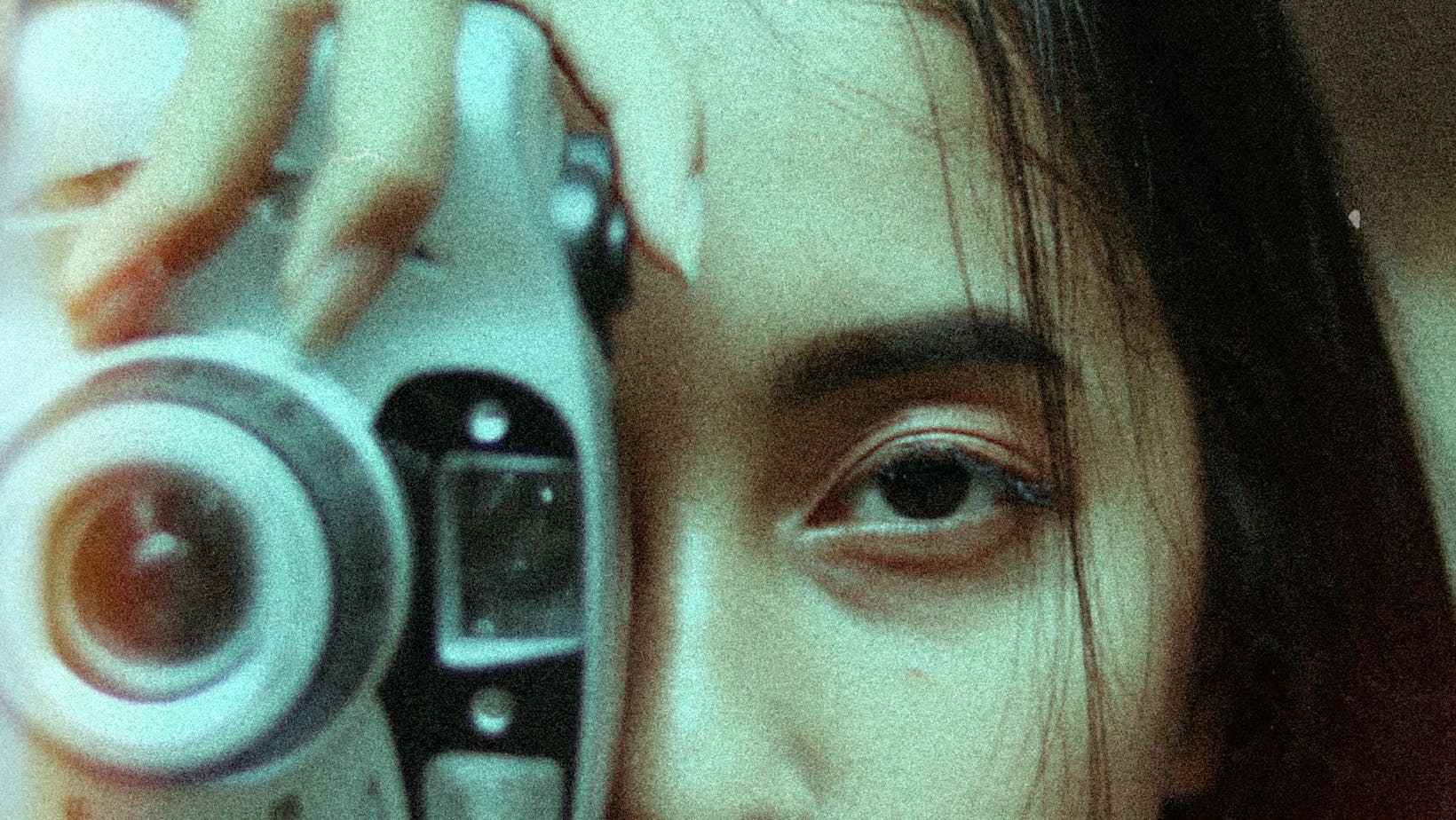
The Role of Sodium Channels
I’d bet my last dollar that if you’ve studied anything related to neuroscience, you’ve come across the term ‘sodium channels.’ They’re pretty big players in this game we call ‘action potential.’ Here’s why.
Sodium channels are like the gatekeepers of your neurons. When a neuron is at rest, these channels aren’t letting any sodium ions through. But when an action potential comes knocking? That’s when the magic happens. These sodium channels open up and let a flood of positive sodium ions rush into the neuron.
This influx causes a surge in electrical charge – it’s what we refer to as ‘depolarization.’ As more and more sodium ions enter, they trigger nearby sodium channels to open as well. It’s almost like setting off a chain reaction or domino effect down the length of the neuron. This wave-like movement of depolarization ensures that an action potential isn’t just a one-and-done event – it regenerates itself along every step of its journey.
Think about it like an applause wave at a sports stadium. One section starts clapping and standing up, then their excitement spreads to the next section, and then to the next, continuing around until everyone’s joined in on this shared phenomenon. That’s exactly how an action potential self-propagates along neurons!
But wait – there’s more! Re-polarization is another crucial process linked with sodium channels. After all those positive charges have rushed in, these channels quickly close again, preventing any further influx while leaving room for potassium ion outflow (thanks to our other pals – potassium channels). This helps re-establish that initial negative resting membrane potential.
So there you have it – Sodium Channels: Gatekeepers extraordinaire! They not only kickstart action potentials by allowing positively charged Sodium ions into neurons but also ensure their propagation down entire lengths of axons through sequential opening and closing. Truly, they’re crucial for our neurons’ communication capabilities.